Why are our piston aircraft engines so @#$%*! inefficient?
Our piston aircraft engines convert chemical energy into mechanical work, but they don’t do it very efficiently. It turns out that only about one-third of the energy contained in the 100LL we burn winds up getting to the propeller and doing useful work to propel us through the air. The remaining two-thirds winds up getting lost between the fuel truck and the prop hub. At today’s stratospheric avgas prices, that’s pretty depressing.
Let’s do the math
Consider a Continental IO-550 engine rated at 300 hp. If the fuel system is set up properly per Continental Service Bulletin SID97-3F, fuel flow at maximum takeoff power is about 26.6 gallons/hour or 156 pounds/hour. How much chemical energy does that fuel provide?
We can calculate that. 100LL is rated at a “minimum lower heat value” of 18,700 BTUs per pound. Let’s convert that figure into something more meaningful to pilots like you and me:
(1) divide 156 pounds per hour by 3,600 seconds per hour to get .0433 pounds per second.
(2) multiply by 18,700 (the thermal content of 100LL in BTUs per pound) to get 810 BTUs per second.
(3) multiply by 1.414 (the horsepower equivalent to one BTU per second) to get 1,146 hp.
Does this mean that your IO-550 consumes 100LL with thermal energy equivalent to 1,322 hp, and yet produces only 300 hp of output power? Unfortunately, that’s EXACTLY what it means—and that works out to a miserable thermal efficiency of 26%. Good grief!
Should an IO-550 really be drinking this much fuel? Well, we can calculate that, too:
(1) At takeoff power, the engine is turning at 2,700 RPM. Since it’s a four-stroke engine, each power cycle requires two crankshaft revolutions. Therefore, the engine is operating at 1,350 power cycles per minute.
(2) The displacement of the engine is 550 cubic inches, or 0.32 cubic feet. Due to induction system losses, however, the engine’s “volumetric efficiency” is only about 85%, so it “inhales” only about 0.27 cubic feet of air per power cycle.
(3) Multiplying 1,350 power cycles per second times 0.27 cubic feet of air per cycle, we calculate that the engine should inhale 365 cubic feet of air per minute.
(4) Sea level air under standard atmospheric conditions weighs 0.0765 pounds per cubic foot. Therefore, the engine breathes 27.9 pounds of air per minute.
(5) Best power mixture requires a air-fuel ratio of about 12.5 to 1 by weight. Dividing 27.9 by 12.5, we get a fuel burn of 2.23 pounds of fuel per minute—or multiplying by 60, we get 134 pounds per hour or 22.3 gallons per hour calculated fuel flow at best power mixture.
The actual book fuel flow figure of 26.6 gallons/hour or 156 pounds/hour is higher than this calculated value because of the unusually rich mixture required to provide adequate detonation margins at full takeoff power.
What about LOP?
Surely engine efficiency is much better at cruise power settings with aggressively lean mixtures, right? Let’s take a look. An IO-550 engine running at 65% power and operating LOP uses approximately 13 gallons/hour or 78 pounds/hour. What kind of thermal efficiency does that represent? Repeating the calculations:
(1) divide 78 pounds per hour by 3,600 seconds per hour to get .0217 pounds per second.
(2) multiply by 18,700 (the thermal content of avgas in BTUs per pound) to get 405 BTUs per second.
(3) multiply by 1.414 (the horsepower equivalent to one BTU per second) to get 573 hp.
So even at LOP cruise, the IO-550 consumes 573 hp worth of go-juice in order to produce 195 hp (65% of 300), for an efficiency of about 34%. Definitely better, but certainly nothing to write home about.
Why so wasteful?
Here’s one breakdown of efficiency losses (from Performance of Light Aircraft by John T. Lowry, Ph.D.):
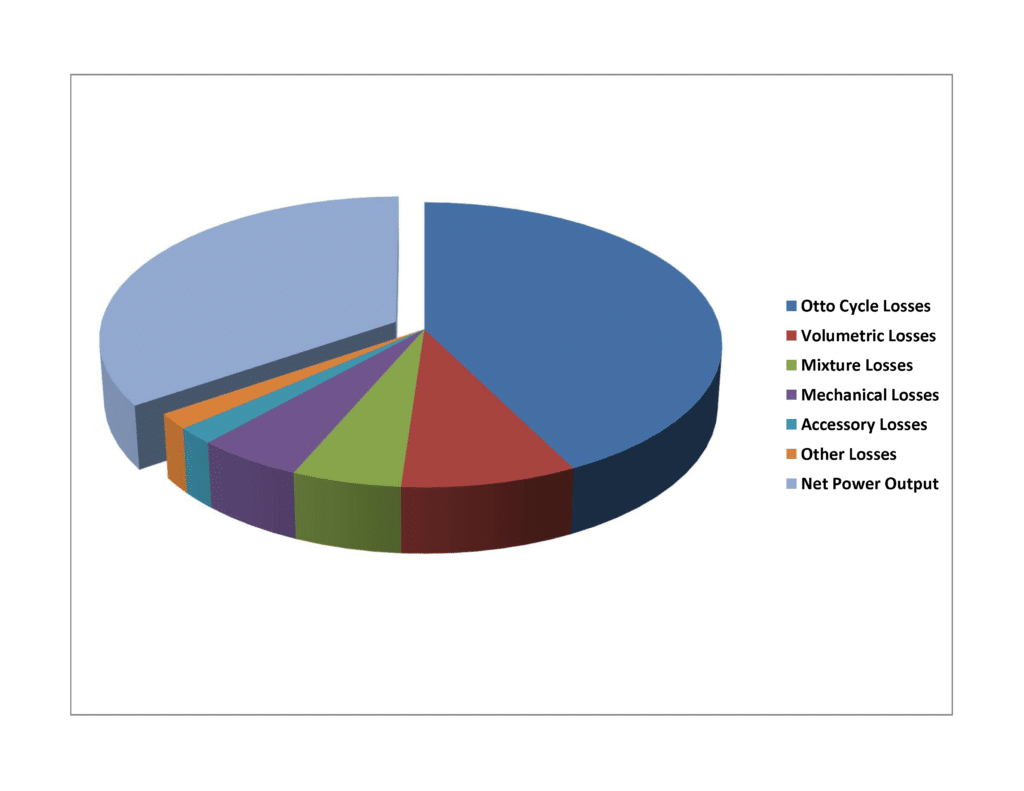
Otto cycle efficiency—the thermodynamic efficiency of a four-stroke internal combustion engine is limited by the compression ratio (i.e., the ratio of cylinder volumes as the piston moves from bottom-dead-center to top-dead-center). The higher the compression ratio, the greater the efficiency. For an IO-550 with a compression ratio of 8.5:1, the Otto-cycle efficiency works out to about 57.5%.
Volumetric efficiency—As mentioned earlier, the ability of the engine to breathe in its full theoretical displacement of air during each power cycle is restricted by a variety of pressure losses at various points in the induction system: air filter, throttle body, intake manifold, and intake valves. For most of our engines, volumetric efficiency is around 85%, bringing total efficiency down to 57.5% x 85% or 49%.
Mixture losses—Optimum fuel efficiency occurs at very lean mixture settings (so-called “best economy mixture”) with an air-fuel ratio in the vicinity of 18-to-1 by weight. Best economy mixture occurs very LOP, however, and most pilots don’t operate that lean. (Not to mention that many engines won’t run smoothly that lean.) Many pilots operate rich of peak EGT in the vicinity of best-power mixture, at an air-fuel ratio around 12.5-to-1, which provides a fuel efficiency that’s only 70% of optimum. Even if you operate slightly LOP (let’s say at an air-fuel ratio of 16-to-1), your efficiency is just 89% of optimum, and that brings total efficiency down to 49% x 89% or 44%.
Mechanical losses—Friction losses involving the reciprocating and rotational parts inside the engine consume a significant amount of power that could otherwise be delivered to the propeller. Mechanical efficiency varies with engine speed (lower losses at lower RPM), but is typically around 88%, bringing total efficiency down to 44% x 88% or 38%.
Accessory losses—A certain amount of engine power is consumed driving accessories such as magnetos, fuel pumps, alternators, vacuum pumps, hydraulic pumps, air conditioning compressors, etc. Figure this robs 5% of the remaining power, bringing total efficiency down to 36%.
Other losses—This includes a grab bag of other inefficiencies including blow-by past the piston rings, unburned hydrocarbons in the fuel, humidity in the air, backpressure in the exhaust system, and so forth. Figure another 5% loss, bringing total efficiency down to 34% (which agrees with our earlier figure for an IO-550-B at 65% LOP).
Thermal and chemical losses
A quite different analysis (from Fundamentals of Power Plants for Aircraft by Joseph Liston) analyzes the various thermal and chemical losses suffered by a piston aircraft engine.
We’ve already seen that an internal-combustion engine is incapable of converting all the heat of combustion into mechanical energy, limited primarily by its finite compression ratio. The rest of the heat of combustion, as well as a small amount of additional heat generated by friction, is lost through the engine’s exhaust and cooling systems.
There are also some chemical losses. In theory, the combustion of pure hydrocarbon fuel at stoichiometric mixture should produce nothing but carbon dioxide (CO2) and water (H2O). In reality, however, there’s always some sulfur in the fuel, which is transformed by combustion to sulfur dioxide (SO2) and sulfuric acid (H2SO4). If the mixture it a bit on the rich side, the exhaust also contains carbon monoxide (CO) which results from incomplete combustion, as well as some unburned carbon particles and some methane gas (CH4).
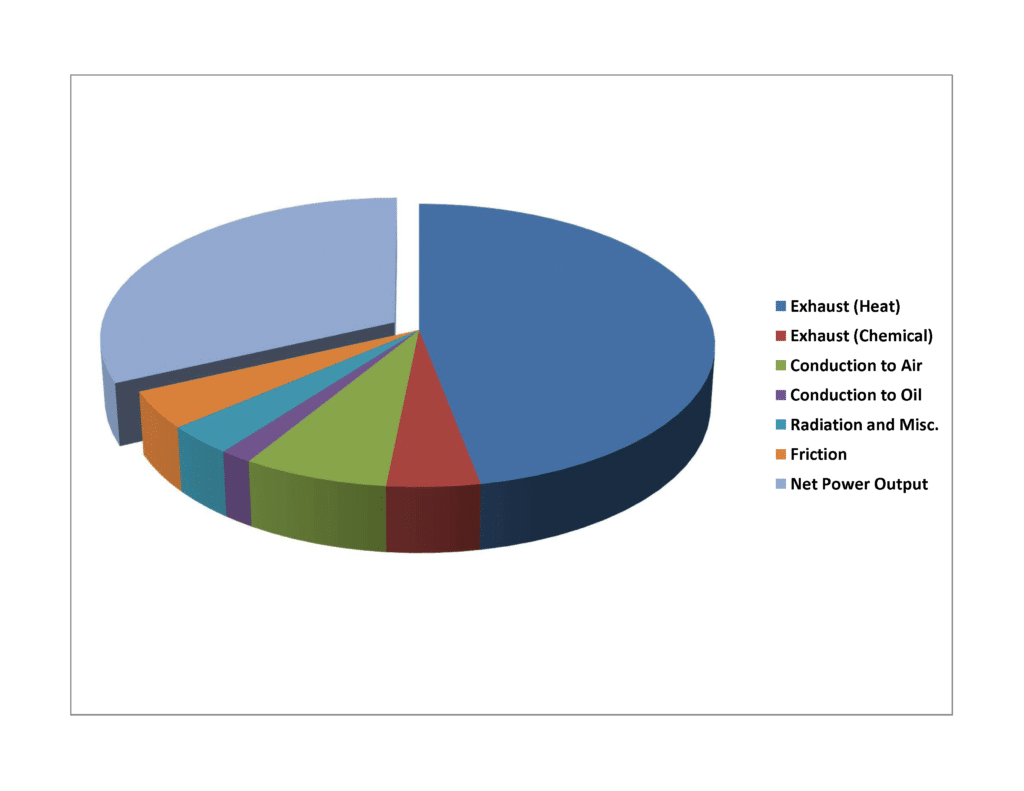
Here’s how Liston breaks this all down:
Fuel energy, 100%
Exhaust, 51.6%
- Heat, 47.0%
- Chemical, 4.6%
- CO, 3.1%
- CH4, 1.5%
Other thermal, 12.2%
- Conduction to air, 7.2%
- Conduction to oil, 1.6%
- Radiation and misc., 3.4%
Mechanical, 36.2%
- Friction losses, 4.3%
- Brake horsepower output, 31.9%
Again, this figure agrees pretty well with our earlier 34% figure for the IO-550-B at 65% LOP cruise.
Can we do better?
What, if anything, can we do to improve this dismal efficiency? Well, don’t expect any miraculous improvements of large magnitude. But every little bit helps, and there are certainly a few areas where the potential exists for improvement:
Otto cycle efficiency—As we’ve seen, the basic thermodynamic efficiency of an internal combustion engine is a function of compression ratio. Unfortunately, high-compression engines have traditionally required high-octane gasoline in order to avoid detonation, and high-octane gasoline is fast becoming unobtainable because of the campaign to eliminate tetraethyl lead (TEL) from avgas. Consequently, the trend in recent years has been toward lower compression ratios that are compatible with low-lead or unleaded fuel. While this may be wonderful for the environment, it sure doesn’t help the thermodynamic efficiency of our engines.
One bright light on the horizon is the prospect of moving from fixed-timed magnetos to sophisticated, computerized electronic ignition systems capable of protecting engines against detonation by varying ignition timing. The incorporation of variable ignition timing and detonation sensors should permit the use of higher compression ratios even with unleaded fuel. It may take a few more years before any such systems make it through FAA certification, but the prospects for improved efficiency are significant.
Even more exciting is the recent advent of certificated Diesel engines for piston aircraft, which run on Jet A and have 18:1 compression ratios that offer much greater thermal efficiency than any spark ignition gasoline engine.
Volumetric efficiency—Small improvements in this area are possible through the use of tuned induction systems, large intake valves, venturi-style valve seats, ram recovery airscoops, and turbocharging. Auto engines have even gone to multiple intake valves per cylinder, but the weight and complexity might make this impractical for aircraft engines.
Mixture losses—Major strides have already been made in this area, partially through pilot education to encourage the use of lean mixture settings, and partially through improvements to engine instrumentation and mixture distribution to facilitate operation at or near best economy mixture (i.e., considerably LOP).
Mechanical losses—The biggest thing that can be done to reduce mechanical losses is for pilots to cruise at low RPM and high manifold pressure, rather than vice-versa. Small additional gains are possible through the use of high-lubricity synthetic oil to reduce friction losses, but the leading all-synthetic oil (Mobil AV 1) was pulled off the market in the 1990s due to its inability to control lead deposits, and even semi-synthetics like Aeroshell 15W-50 have lead-deposit problems, particularly in small-sump engines like the ones used in the Cessna TTX and Cirrus SR22. When the lead is ultimately removed from avgas, all-synthetic oils may one come back in favor for piston aircraft engines.
Accessory losses—The conversion to electronic ignition systems may also provide some small benefits by eliminating the mechanical losses involved in driving dual magnetos, although this may be partially offset by the requirement for electronic-ignition engines to have dual alternators. The trend toward all-electric airplanes with no pneumatics or hydraulics may also help slightly.
For now, the best thing you can do to improve efficiency is to lean aggressively (considerably LOP if feasible), and to cruise at low RPM and high MP rather than vice-versa. In the foreseeable future, further improvements may be possible through the use of variable-timing electronic ignition systems and installation of higher-compression pistons. Efficiencies in the area of 40% are possible, but don’t expect much more than that from spark-ignition engines, at least any time soon.
You bought a plane to fly it, not stress over maintenance.
At Savvy Aviation, we believe you shouldn’t have to navigate the complexities of aircraft maintenance alone. And you definitely shouldn’t be surprised when your shop’s invoice arrives.
Savvy Aviation isn’t a maintenance shop – we empower you with the knowledge and expert consultation you need to be in control of your own maintenance events – so your shop takes directives (not gives them). Whatever your maintenance needs, Savvy has a perfect plan for you: