There’s a lot more to engine bearings than meets the eye.
According to Miriam-Webster, a bearing is “a machine part in which another part turns.” Most aircraft have lots of them.
Wheels spin on their axles with the help of tapered roller bearings. Magnetos, alternators, generators and starter motors incorporate ball bearings to support their rotors. The landing gear trunions on my Cessna 310 pivot on needle bearings. Variable-pitch propeller blades are supported by large-diameter ball bearings. Turbine engine rotor shafts spin in ball and roller bearings. All these bearings consist of inner and outer “races” with spherical or cylindrical rolling elements between them. Such “rolling-element bearings” do a superb job of supporting a shaft in precise position while permitting it to rotate with very little friction.
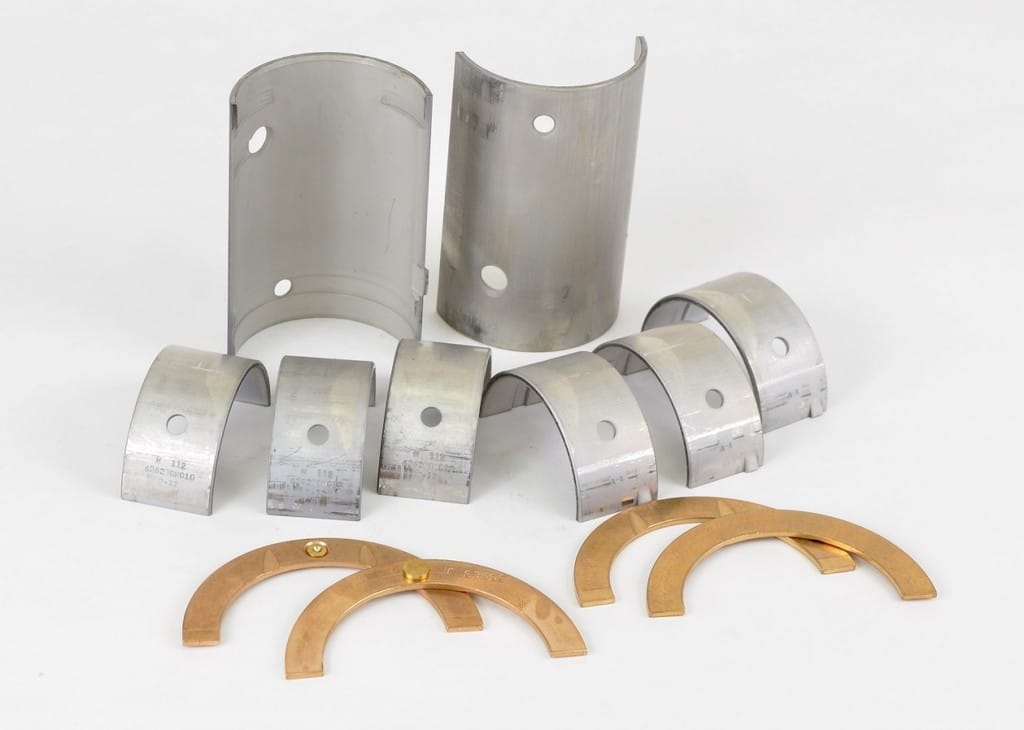
But tear down a Continental or Lycoming engine and you won’t bearings like those. The bearings in which the crankshaft, crankpins, camshaft, rocker shafts and piston pins run have no races, balls, rollers, needles or other moving parts. They’re just curved pieces of metal—known variously as “plain bearings” or “sleeve bearings” or “bushings”—that rely on sliding elements rather than rolling ones. Plain bearings are usually constructed of two semicircular halves called “shells”; one-piece plain bearings are usually called “bushings.”
There’s a good reason that reciprocating engines use plain bearings: They reciprocate! This means that the crankshaft, crankpin, piston pin, rocker shaft and camshaft bearings are subject to continuous sharp cyclic loads. Rolling-element bearings don’t handle such loads very well, because they concentrate loads into very tiny contact regions between the rolling elements and the races, resulting in extremely high pressures. If ball bearings were used in a reciprocating engine, the result would be peened races, flat-spotted balls, and consequent short bearing life.
By contrast, plain bearings tolerate such cyclic loads better because they distribute the loads over a much larger area, so the pressure is greatly reduced. They also do a better job of accommodating shaft flexing, minor misalignments, and wide temperature swings. That’s why nearly all reciprocating engines—from one-cylinder motorcycle engines to giant marine diesels—use plain bearings instead of ball or roller bearings.
These plain bearings and bushings look simple, but they aren’t. There’s a lot more to them than meets the eye.
Lubrication
When I had the engines in my Cessna 310 torn down for overhaul in 1990, I made a point of paying a visit to the engine shop to survey the damage before the engine was put back together. The engines had accumulated 1900 hours over 11 years, and I remember being rather astonished at the appearance of the main and rod bearings; they looked nearly brand new!
How can a plain bearing with no rolling elements withstand the torturous environment of a high-performance turbocharged reciprocating aircraft engine for 1900 hours and 11 years without showing any significant signs of wear? There’s a two-word answer: hydrodynamic lubrication.
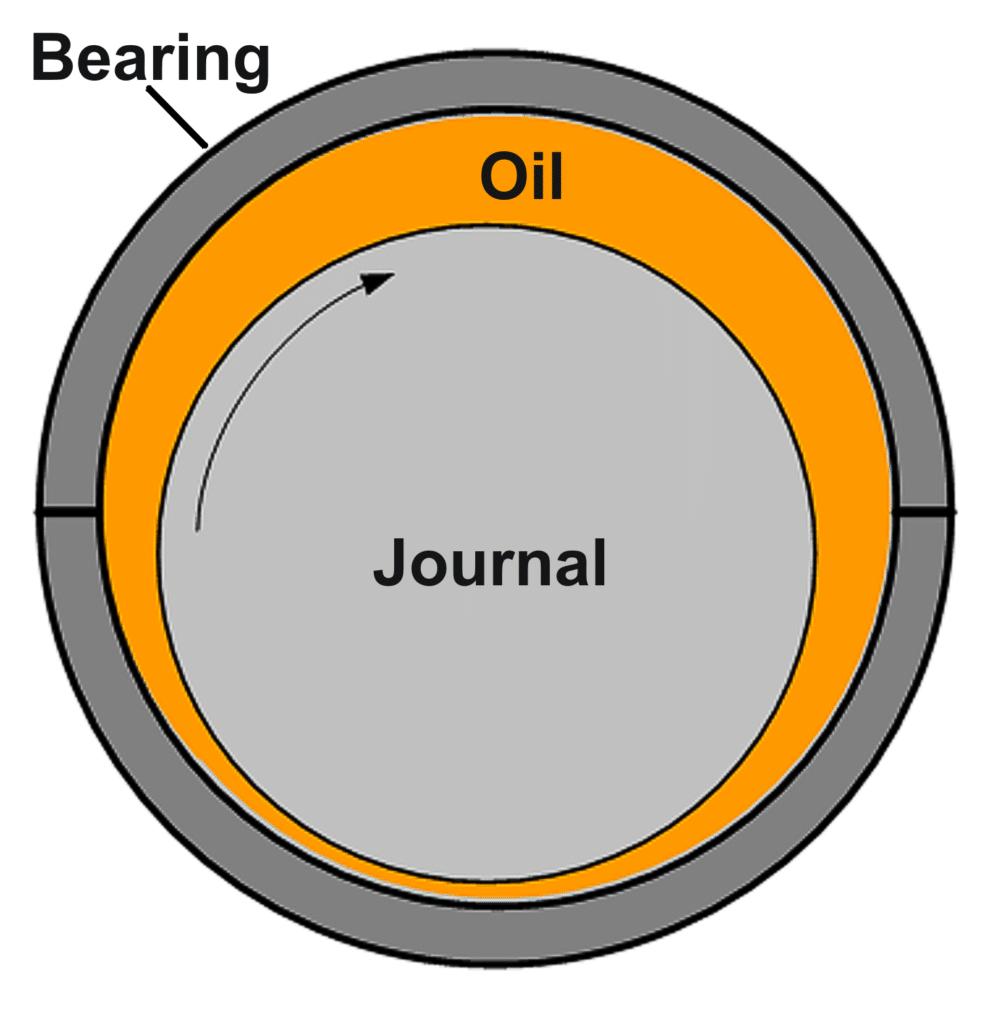
Plain bearings rely on hydrodynamic lubrication to prevent metal-to-metal contact between the rotating journal and the stationary bearing. (See Figure 2.) Pressurized oil is pumped continuously into the gap between the journal and the bearing. This gap is only about .002” wide—about the thickness of a human hair. Rotation of the journal within the bearing, together with the viscosity of the oil, creates a dynamic wedge of high-pressure oil that keeps the parts separated. So long as the bearing gets adequate oil pressure and the journal rotates rapidly enough, there is no metal-to-metal contact and therefore no wear on either the bearing or the journal.
Does this mean that plain bearings can last forever? Actually yes, provided the engine is run continuously (as it might be in a test cell) with an uninterrupted oil supply and uninterrupted journal rotation. Unfortunately, that’s not what happens in the real world. We start up our engines, run them for an hour or three while we’re flying from point A to point B, and then shut them down. It’s mainly those pesky startups and shutdowns that limit the useful life of plain bearings.
When we first crank the engine, there’s no oil pressure and the crankshaft cranking speed is pathetically slow. The conditions for hydrodynamic lubrication simply do not exist. Consequently there is metal-to-metal contact between the journal and the bearing, and wear is inevitable.
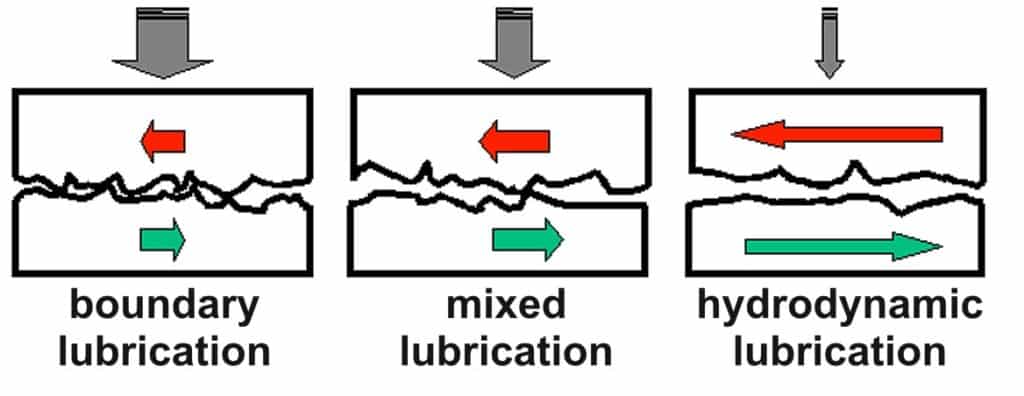
This startup wear is mitigated in several ways: The mating journal and bearing surfaces are polished as smooth as possible. The bearing surface is made of a material that has low sliding friction against the steel journal. Anti-wear additives in the oil react with the metal to form a thin protective film on the surfaces that further reduce friction through a phenomenon known as “boundary lubrication.” (See Figure 3.)
Getting oil to the bearings
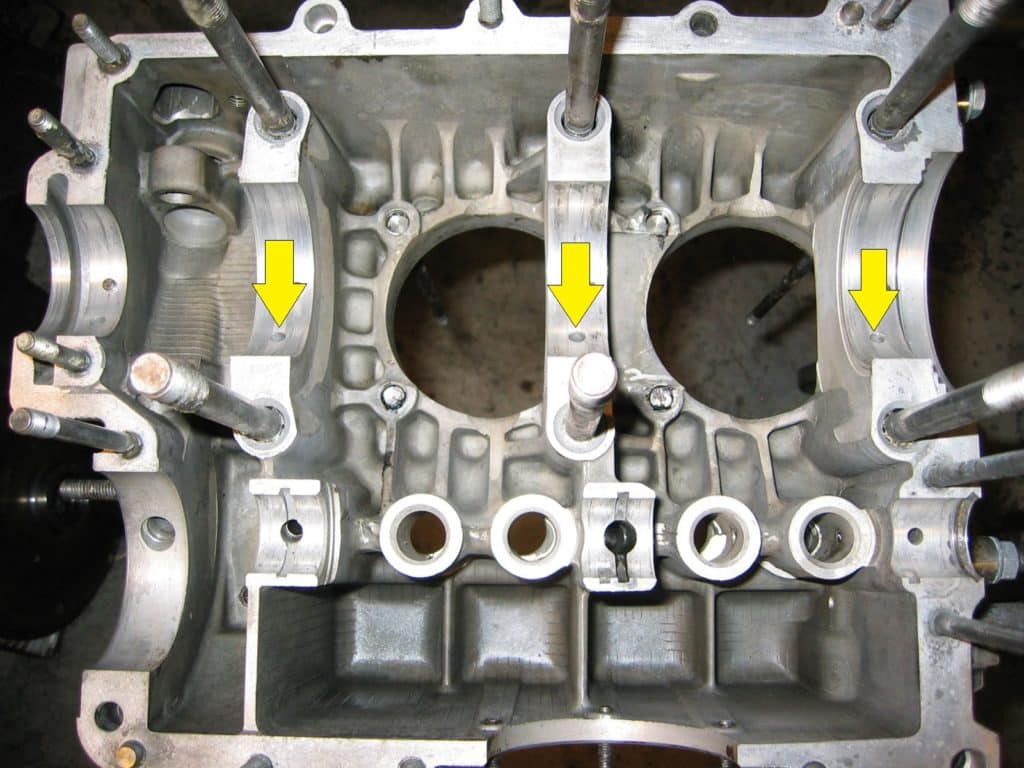
Once the engine is running, pressurized oil passes through “galleries” (often misspelled “galleys”), which are internal passageways machined into the crankcase halves to conduct oil to key components such as main bearings and hydraulic lifters. Oil passages in each main bearing saddle connect to the galleries. (See Figure 4.) Each main bearing shell has an oil supply hole that lines up with the oil passage in the saddle.
Getting oil to the crankpin bearings in the big ends of the connecting rods is a bit trickier. The crankshaft is machined with diagonal passages to conduct oil from the main bearings to the crankpin journals. (See Figure 5.)
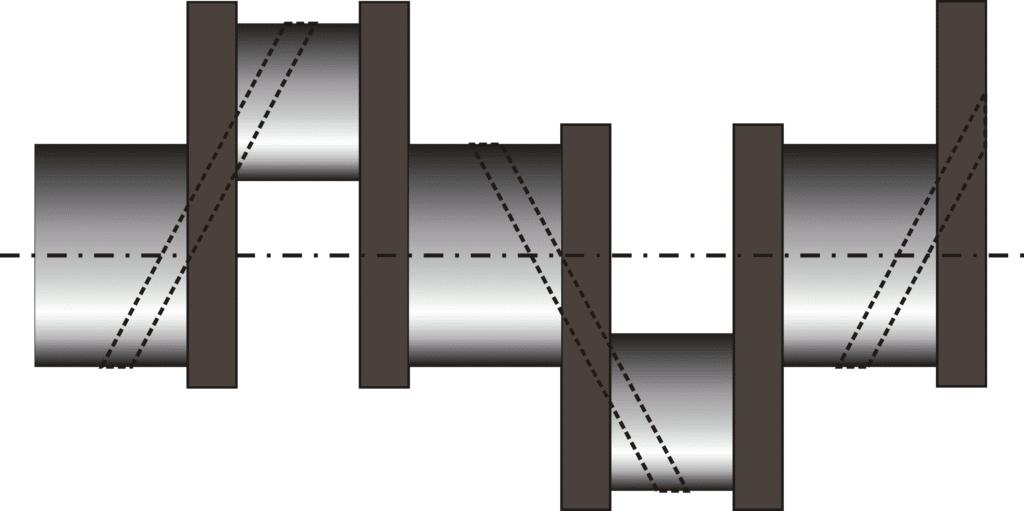
Oil pumped into the main and rod bearings is extruded from the edges of the bearings and returns to the engine’s oil sump or tank. The rapidly rotating crankshaft flings this oil in all directions, filling the crankcase with a dense oil mist that provides “splash lubrication” to other engine components like the cam lobes, lifter faces, piston pin bushings and cylinder barrels.
Material properties
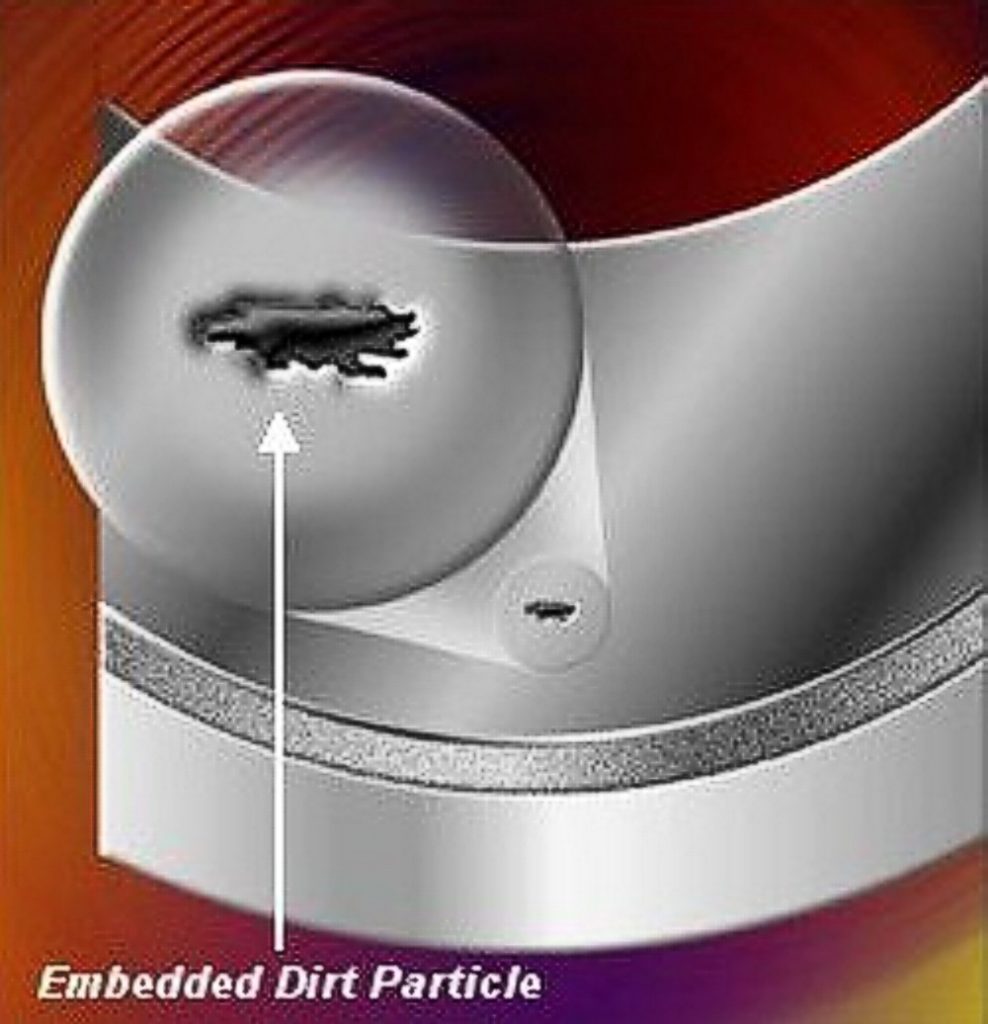
Plain bearings must have a running surface that will slide against steel journals with low friction and be highly resistant to galling (i.e., adhering). Because the bearing is exposed to high cyclic loads and high temperatures, its running surface must have good fatigue strength and retain that strength under high heat. It also needs to be resistant to corrosive attack by moisture and acids. Yet another key property is called “embeddability” and means that the bearing’s running surface is soft enough to allow small particles of dirt, metal or other foreign material to become embedded in the bearing so it doesn’t scratch the crankshaft journal or jam the tiny oil clearance zone between the journal and the bearing. (See Figure 6.)
Most bearings in piston aircraft engines have a running surface made of a family of alloys known as “babbitt” or “white metal” that are about 90% tin combined with small quantities of antimony and copper. (The term “babbitt” derives from Isaac Babbitt, who invented the original version of this bearing alloy in 1839 in Taunton, Massachusetts.) Babbitt offers exceptional slipperiness and embeddability, but its fatigue resistance and temperature strength deteriorate rapidly if more than a few thousandths of an inch thick.
Trimetal bearings
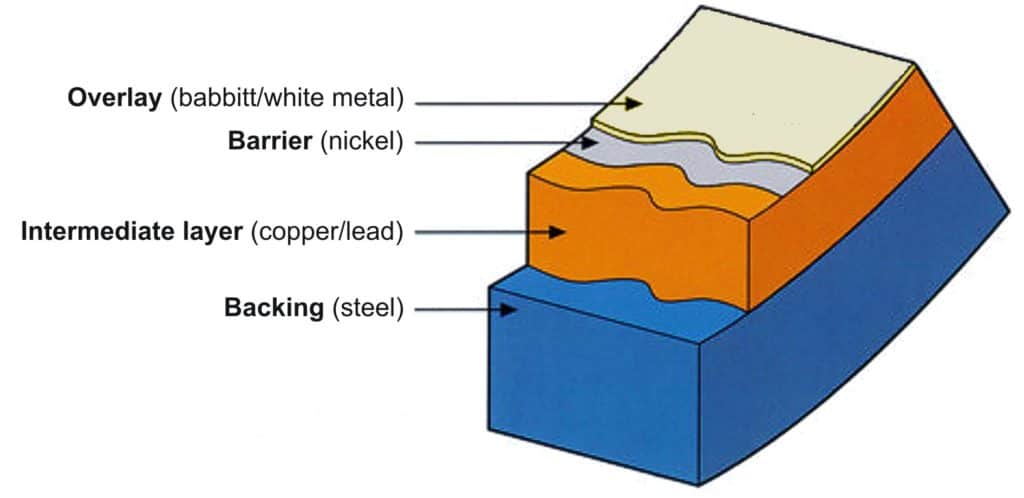
Consequently, most piston aircraft engine bearings use a layered construction referred to as “trimetal” although they actually have four or five layers. (See Figure 7.)
The bearing shell starts out with a semicircular steel backing, to which is bonded an intermediate layer of copper/lead alloy designed to provide the necessary cushioning, fatigue strength and temperature conduction. A thin overlay of babbitt is then electroplated over the intermediate layer in order to provide the required surface properties (slipperiness, embeddability, corrosion-resistance). A micro-thin layer of nickel is deposited between the intermediate layer and overlay in order to prevent tin from migrating from the babbitt into the copper/lead alloy. Sometimes, another micro-thin layer of pure tin is deposited on top of the babbitt overlay to protect it from corrosion prior to installation in the engine.
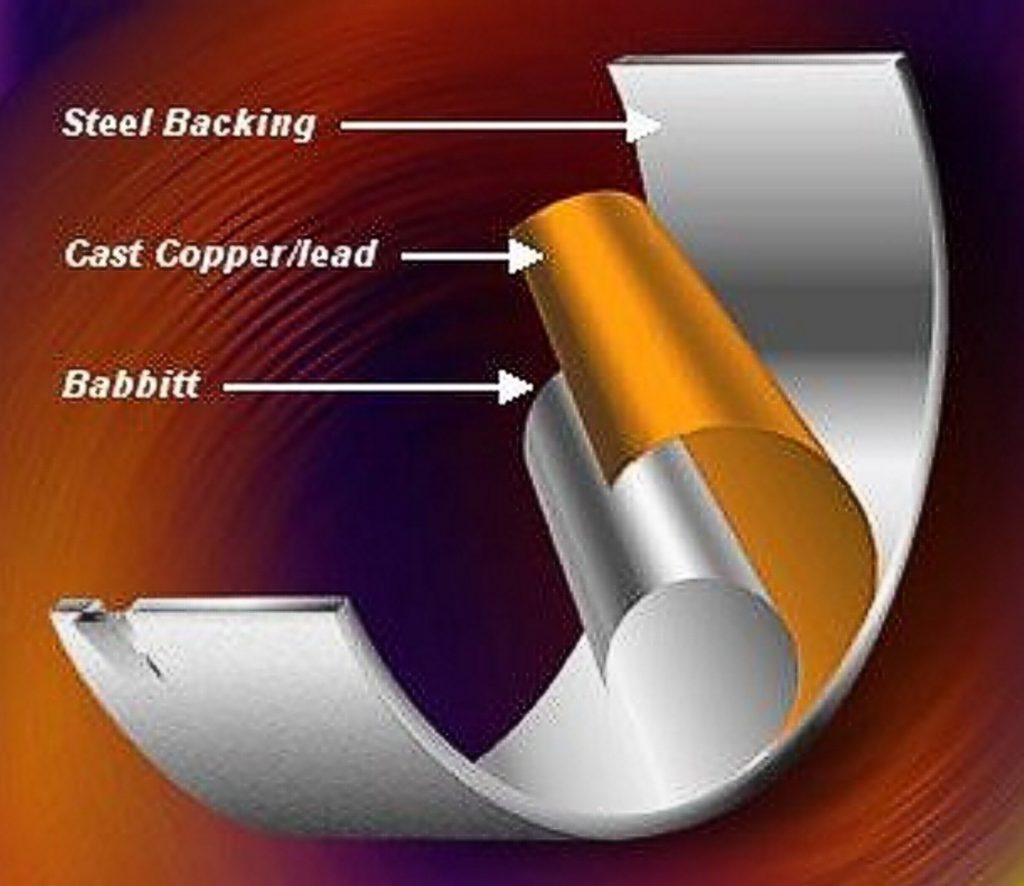
It can be useful to think of the construction of a trimetal bearing as being like a bed: The steel backing is like the box spring (providing support), the intermediate layer is like the mattress (providing cushioning), and the overlay is like a silk sheet (thin, smooth, slippery and comfortable).
The surface properties (slipperiness and embeddability) of the copper/lead intermediate layer are not nearly as good as those of the babbitt overlay, but they’re generally adequate to prevent the bearing from self-destructing suddenly in the event the overlay is worn away. If that happens, the engine will start “making copper” that will be apparent in oil analysis and (if it gets bad enough) as visible nonferrous metal in the oil filter. That means it’s teardown time.
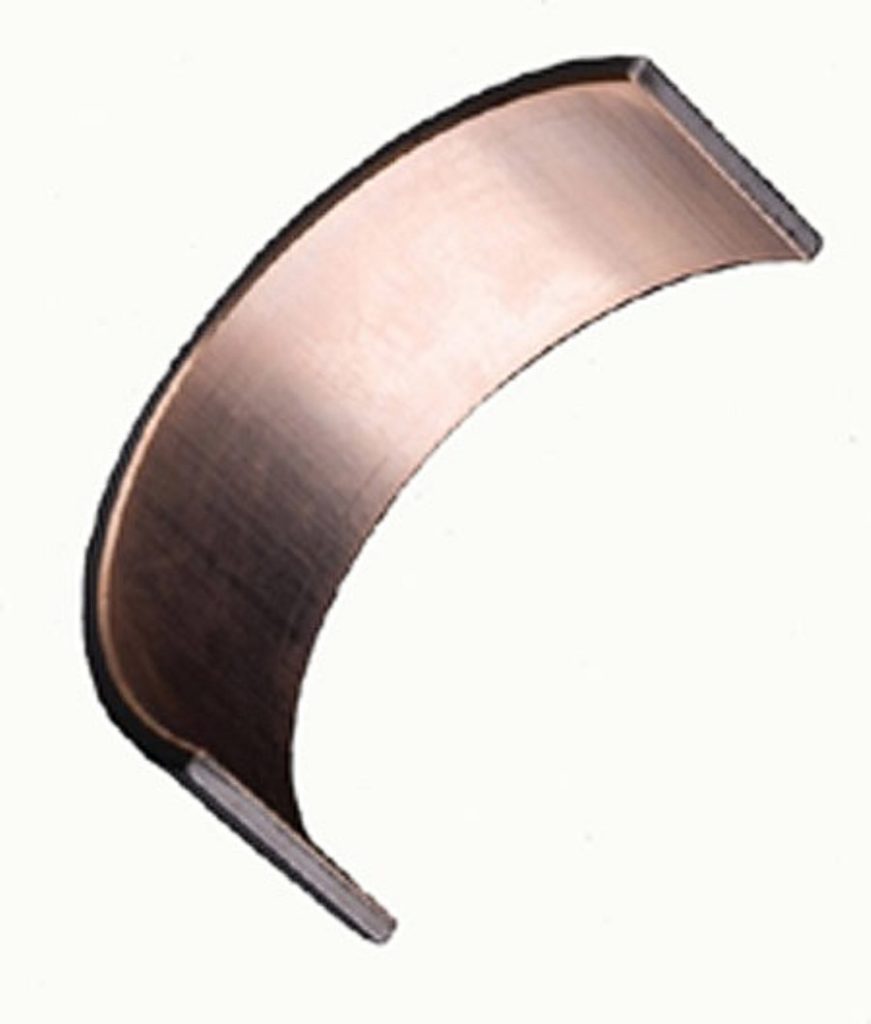
NOTE: During the period 1995 through 2001, Lycoming used bearings with an intermediate layer composed of aluminum/tin alloy instead of copper/lead alloy. These aluminum bearings proved to have lower fatigue strength and a higher failure rate, so Lycoming stopped using them in February 2002.
Securing the bearings
The trimetal bearing shells are mounted in semicircular “saddles” in the crankcase, and secured firmly in place by means of a preload referred to as “bearing crush.” As shown in Figure 10, the steel backing of the bearing shell is slightly taller (by a few thousandths of an inch) than the crankcase saddle in which the shell is installed.
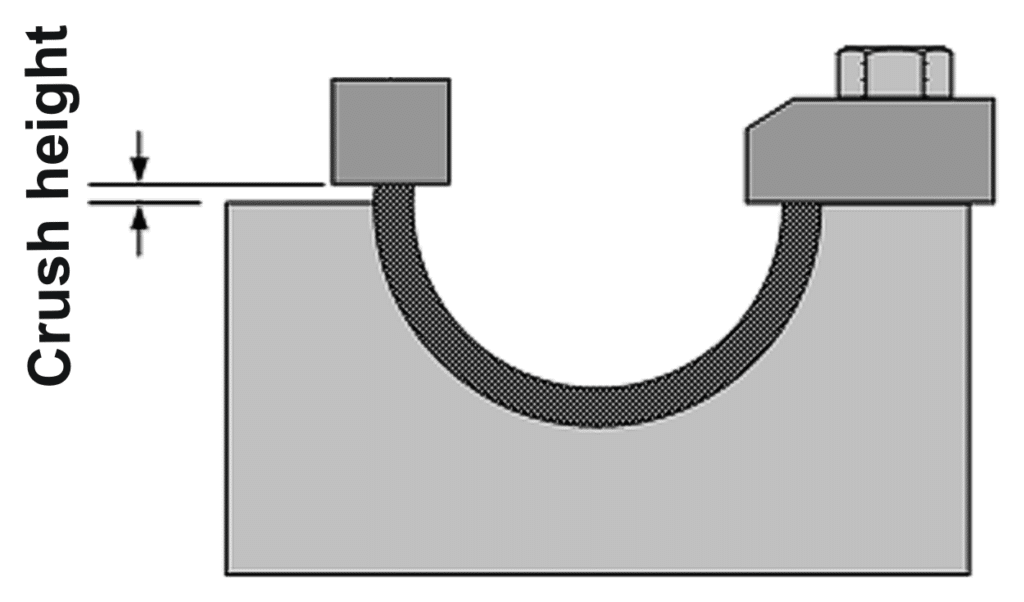
When the engine is assembled and the through-bolts are torqued to spec, the bearing shell is firmly locked into the saddle with an interference fit.
IMPORTANT: If this bearing crush is lost—due to improper crankcase machining at overhaul or improper through-bolt torque during cylinder installation or replacement—the bearing can shift in its saddle, creating misalignment of the bearing’s oil supply hole with the saddle’s oil passage and causing impaired oil flow to the bearing. This condition—referred to as a “spun” or “displaced” bearing—can result in catastrophic engine failure.
Failure modes
Although trimetal bearings usually have excellent longevity when operated within design limits and provided adequate lubrication, premature bearing failure does occur. Figure 11 shows a rod bearing whose babbitt overlay is almost completely worn away, leaving the copper/lead intermediate layer fully exposed.
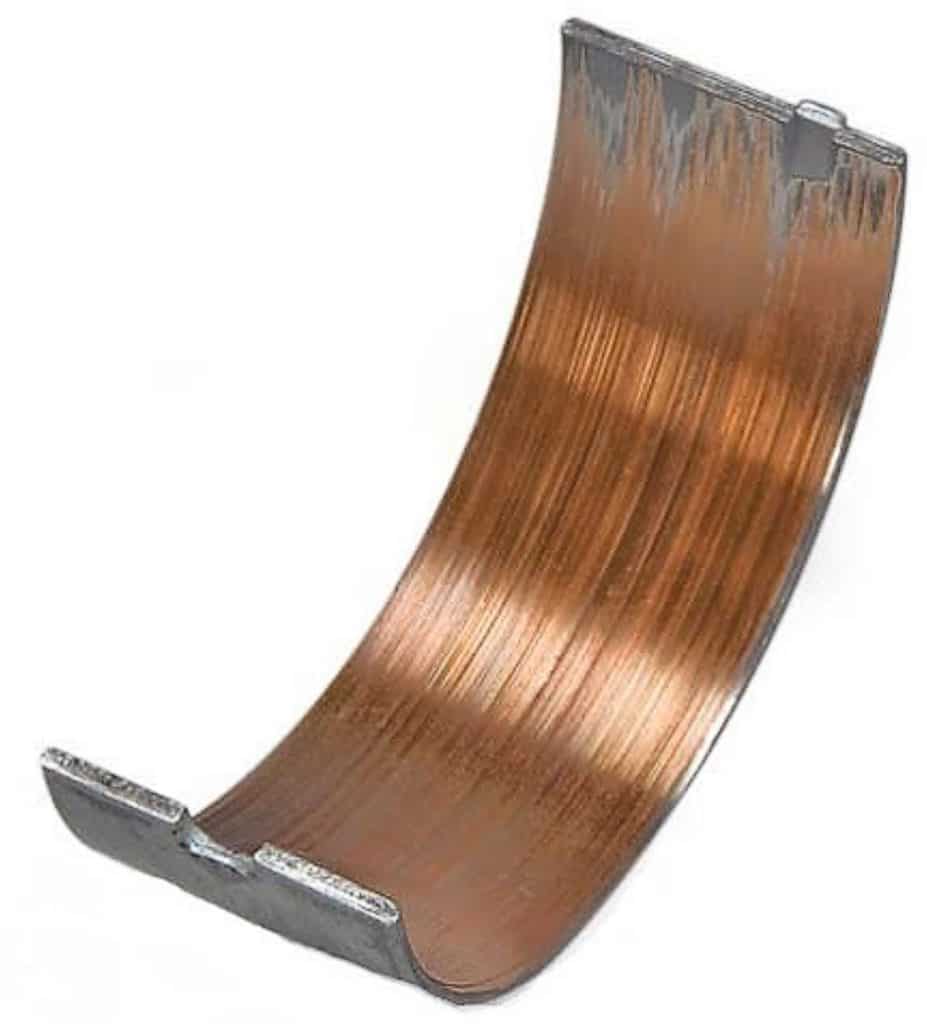
Figure 12 shows a badly contaminated rod bearing with large amounts of foreign material (mostly metal particles) embedded in its babbitt overlay. Catastrophic failure (thrown rod) was probably not far away.
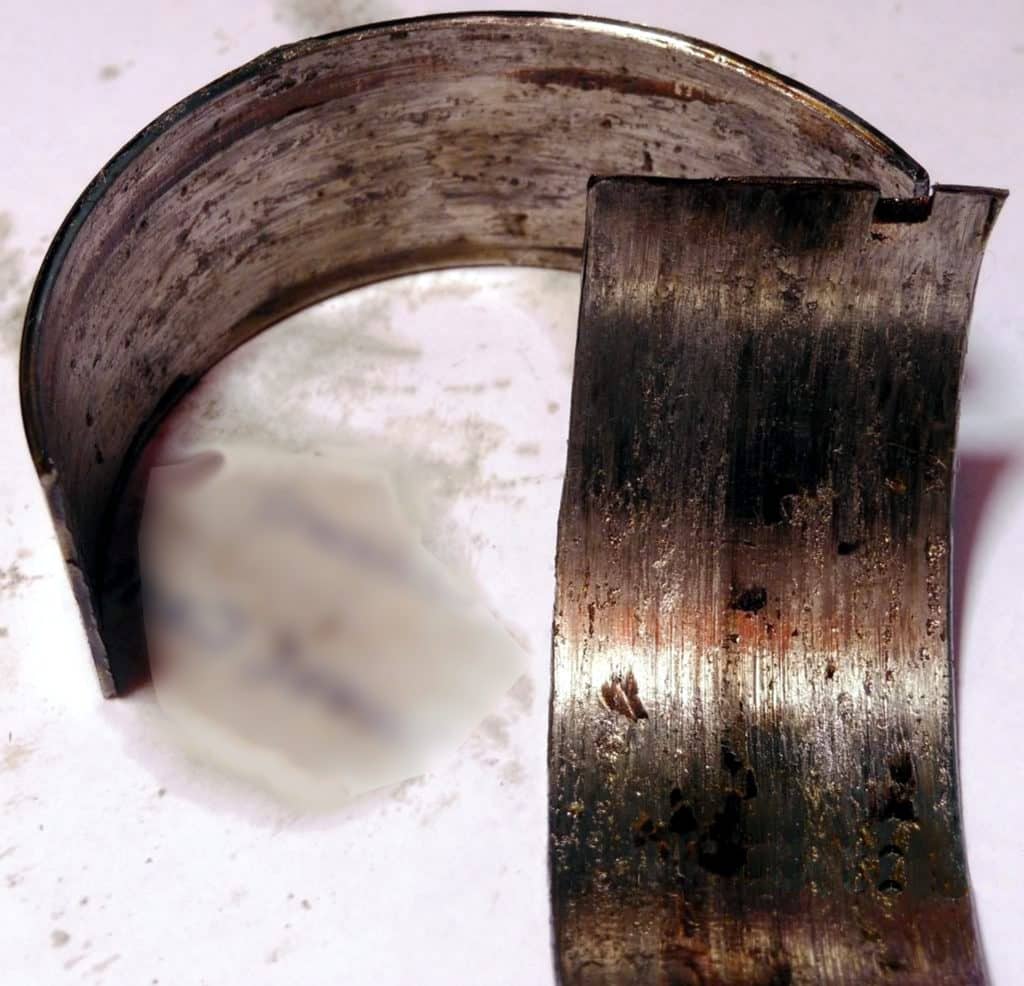
Figure 13 shows another rod bearing exhibiting severe damage due to cyclic overload, most likely destructive detonation or preignition in the associated cylinder. The extreme peak combustion pressures created loads on the connecting rod that exceeded the ability of hydrodynamic lubrication to prevent metal-to-metal contact between the rod bearing and crankpin. Undoubtedly the cylinder head and piston also sustained damage.
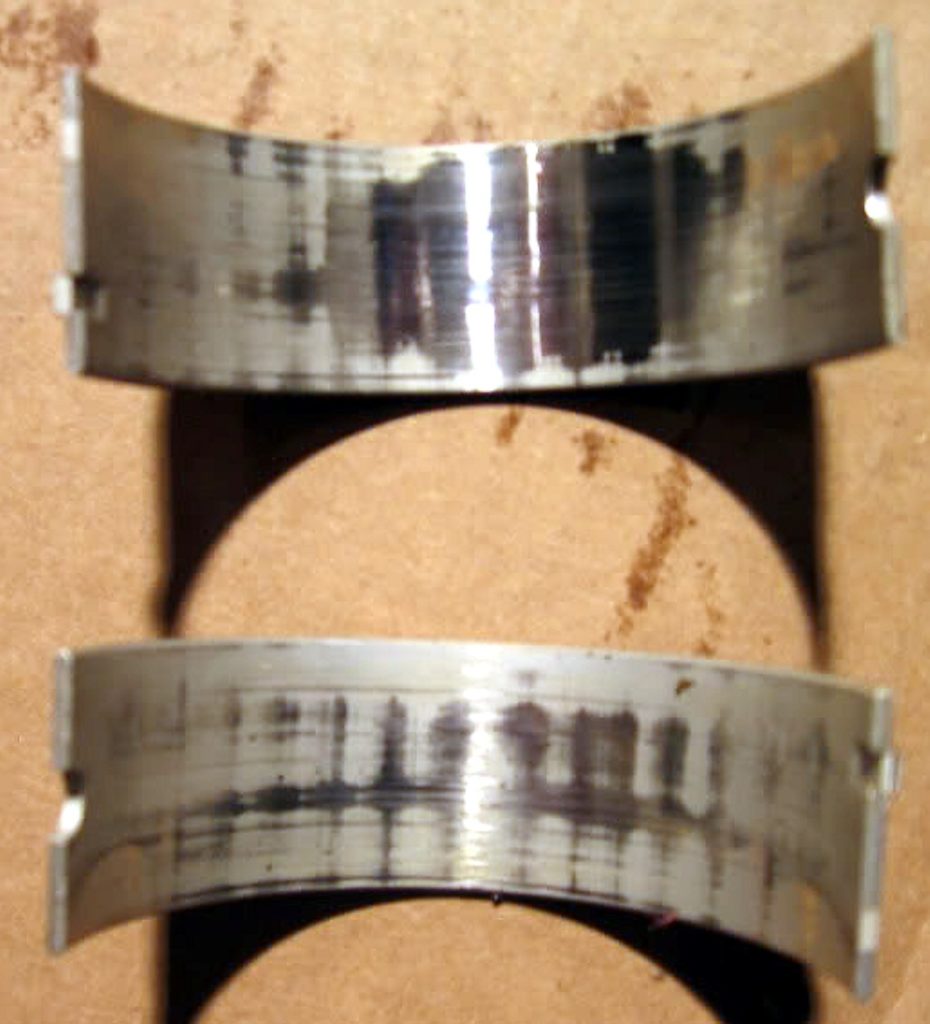
You can tell all of these are rod bearings (rather than main bearings) because they don’t have oil supply holes. As a general rule, rod bearings are more highly stressed and susceptible to damage and failure than main bearings.
The (not so) plain bearings in your piston aircraft engine will generally provide trouble-free service to TBO and often well beyond. (The ones in my Cessna 310’s engines are well past 200% of TBO.) To accomplish that, all they really need a steady supply of clean oil at a decent pressure (to provide good hydrodynamic lubrication), and some anti-wear additives to provide good boundary lubrication at engine start. Contamination is the bearing’s main enemy, so make sure you have a good full-flow oil filter capable of trapping any particulate matter larger than about .001” (25 microns).
Inspect the filter regularly and watch your oil analysis reports for elevated copper or tin. If the filter is clean and the oil report looks normal, then you have nothing to worry about.
You bought a plane to fly it, not stress over maintenance.
At Savvy Aviation, we believe you shouldn’t have to navigate the complexities of aircraft maintenance alone. And you definitely shouldn’t be surprised when your shop’s invoice arrives.
Savvy Aviation isn’t a maintenance shop – we empower you with the knowledge and expert consultation you need to be in control of your own maintenance events – so your shop takes directives (not gives them). Whatever your maintenance needs, Savvy has a perfect plan for you: